By Eric Niderost
Enrico Fermi’s face was a study in concentration as his fingers deftly moved across the well-worn surface of his slide rule. The Nobel-Prize winning physicist instinctively knew this was the moment that would mean the difference between triumphant success and bitter, heartbreaking failure in pursuit of the first sustained nuclear reaction. And yet the Italian-born scientist also exuded a confidence that was a soothing balm to at least some of the more nervous colleagues that clustered around him, peering over his shoulders or craning their necks to see his computations.
The group were perched on a high balcony overlooking C-1, or Chicago 1, a primitive early nuclear reactor that was a major step—if everything worked as planned—toward the development of an atom bomb. The immediate goal here was to create a self-sustaining chain reaction, where waves of neutrons split atoms, the very act of division creating more neutrons that would in turn collide with other atoms. But this atomic “domino” effect had a crucially important byproduct. The splitting of certain kinds of atoms, a process which scientists called “fission,” released energy.
If that energy could be harnessed, contained, and safely controlled, the possibility of nuclear power would thrust humanity into a new age of peril and well as promise. But the year was 1942, World War II was raging on battle fronts across the globe, and the possibility that Hitler and the Nazis might develop an atom bomb before the Allies gave this scientific work a new and sobering reality. This was not just an academic exercise, of little interest to anyone outside a college campus or laboratory. The results that came from this crude Chicago reactor would determine the fate of the Allied cause.
All matter, inanimate or living, is made up of molecules. Molecules in turn are made up of smaller elements called atoms. Atoms are infinitely small, so tiny that one million of them could line up across the breadth of a human hair. By the 1920s, it was realized that each atom has a central core, or nucleus, that is made up of subatomic particles called protons. They have a positive charge. Each nucleus also has negatively charged particles, electrons, swirling around it, orbiting as if the nucleus was a “sun” and they were “planets.”
But the path that led to the atomic bomb began in 1932, when British physicist James Chadwick discovered the nucleus also has particles he dubbed “neutrons.” Opposites attract, so positive protons and negative electrons are “glued” together in a seemingly unbreakable bond. But the neutron is the spoiler, has no charge at all, and so is not “forced” to remain with the other particles.
That fact was the key. Uranium is an unstable element and naturally throws off neutrons over time. If a neutron hit a uranium atom, that atom’s nucleus would fission, or split, creating two smaller atoms, but also two or three more neutrons that would travel on to other uranium atoms to repeat the process. This is known as a chain reaction. But with each collision energy would be released, building and building until it reached an almost unimaged scale. If you fissioned roughly two pounds of uranium, you could create an explosion the equivalent of 20,000 pounds of TNT.
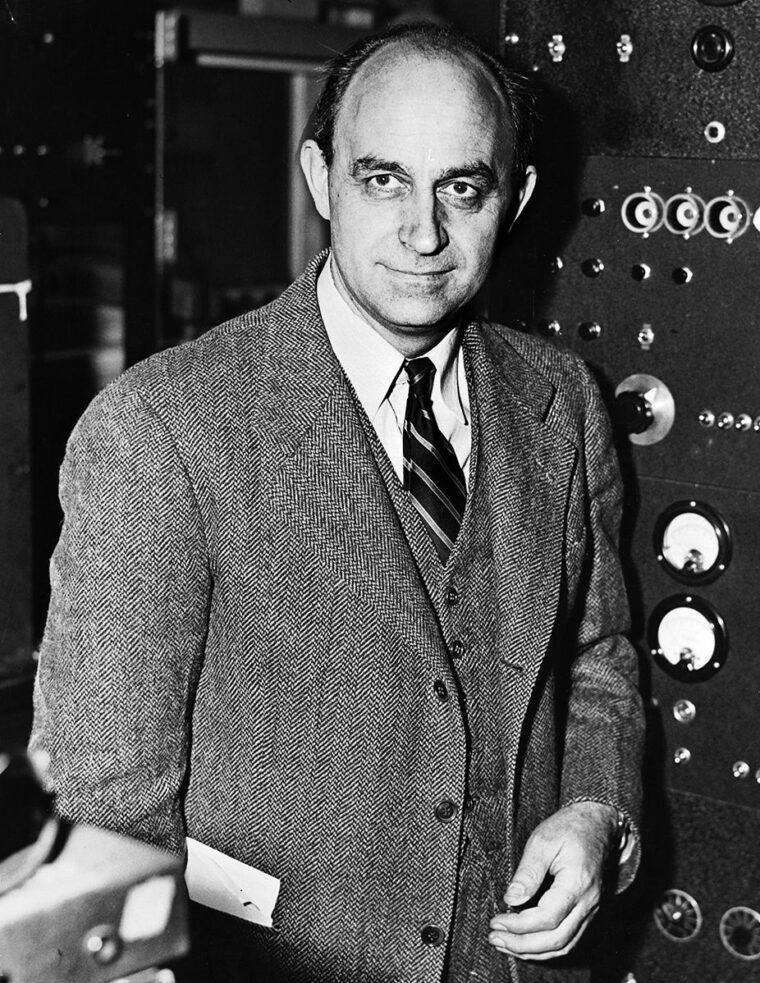
In December 1938, two German physicists, Otto Hahn and Fritz Strassman, working at the Kaiser Wilhelm Institute in Berlin, were puzzled about some test results they were getting. They consulted with fellow scientist Lise Meitner in Sweden. Meitner had been a colleague, but she was Jewish, and with anti-Semitism on the rise in Nazi Germany, wisely decided to emmigrate. It was Meitner who realized what had happened: Hahn and Strassman had achieved uranium fission.
The news spread through the international scientific community and came to the attention of Leo Szilard, a Hungarian who moved to the United States in the 1930s. Brilliant but eccentric, Szilard tried to write to American government officials but was ignored. Hitler was on the march; a few months earlier British and France had appeased the dictator by permitting a dismemberment of Czechoslovakia in the Munich Agreement. Szilard feared time was running out, and something had to be done before the Nazis developed an atom bomb.
Szilard enlisted the aid of a fellow Hungarian, Eugene Wigner, and together they travelled to Long Island to visit the most famous scientist in the world, Albert Einstein. Einstein was a pacifist, but he put aside his qualms, at least for the moment. The trio of scientists agreed they would cut through the red tape and go right to the top by sending a letter to President Franklin Roosevelt. The letter would be Einstein’s officially, and he would sign it, but much of the actual text was composed by Szilard.
The letter, dated August 2, 1939, talked about uranium as a new power source, discussed recent events, then warned, “This new phenomenon would also lead to the construction of bombs.” Einstein and the ghostwriting Szilard weren’t too sure how these superbombs might be delivered to the target; perhaps they might be too heavy for airplanes. But the danger was still there. Einstein went on, “A single bomb, carried by boat, and exploded in a port, would destroy the whole port with some of the surrounding territory.”
Roosevelt was prescient enough to recognize the truths outlined in the missive and ordered preliminary research initiated at once. But funds were lacking or diverted to other, more pressing concerns. The secret development of the atomic bomb, later called the Manhattan Project, didn’t really get off the ground until the United States entered the war after Pearl Harbor. By July 1942, the project was given a new sense of purpose and a welcome boost in efficiency when it was placed under the control of The U.S. Army Corps of Engineers. General Leslie R. Groves was tapped to administer every phase of the development work.
Professor Arthur Compton, Nobel Prize winning physicist at the University of Chicago, gathered scientists who would be of use on building a reactor that could produce a sustained and controlled nuclear chain reaction. Enrico Fermi of Columbia University in New York would head this effort. Fermi was a political refugee who could not stomach the fascism of Italy’s Benito Mussolini. Outwardly he was an ordinary looking man, with thick eyebrows and a receding hairline, but appearances were deceiving.
There was a brilliant mind underneath the bald pate, and he was renowned for his surprising physical strength and stamina. It was said even extremes of temperature could not faze him; he once challenged some younger scientists to a tennis match under a broiling, humid summer sun. After an hour or more, the younger men were wilting and ready to throw in the towel. Fermi, astonished, commented on their lack of vigor.
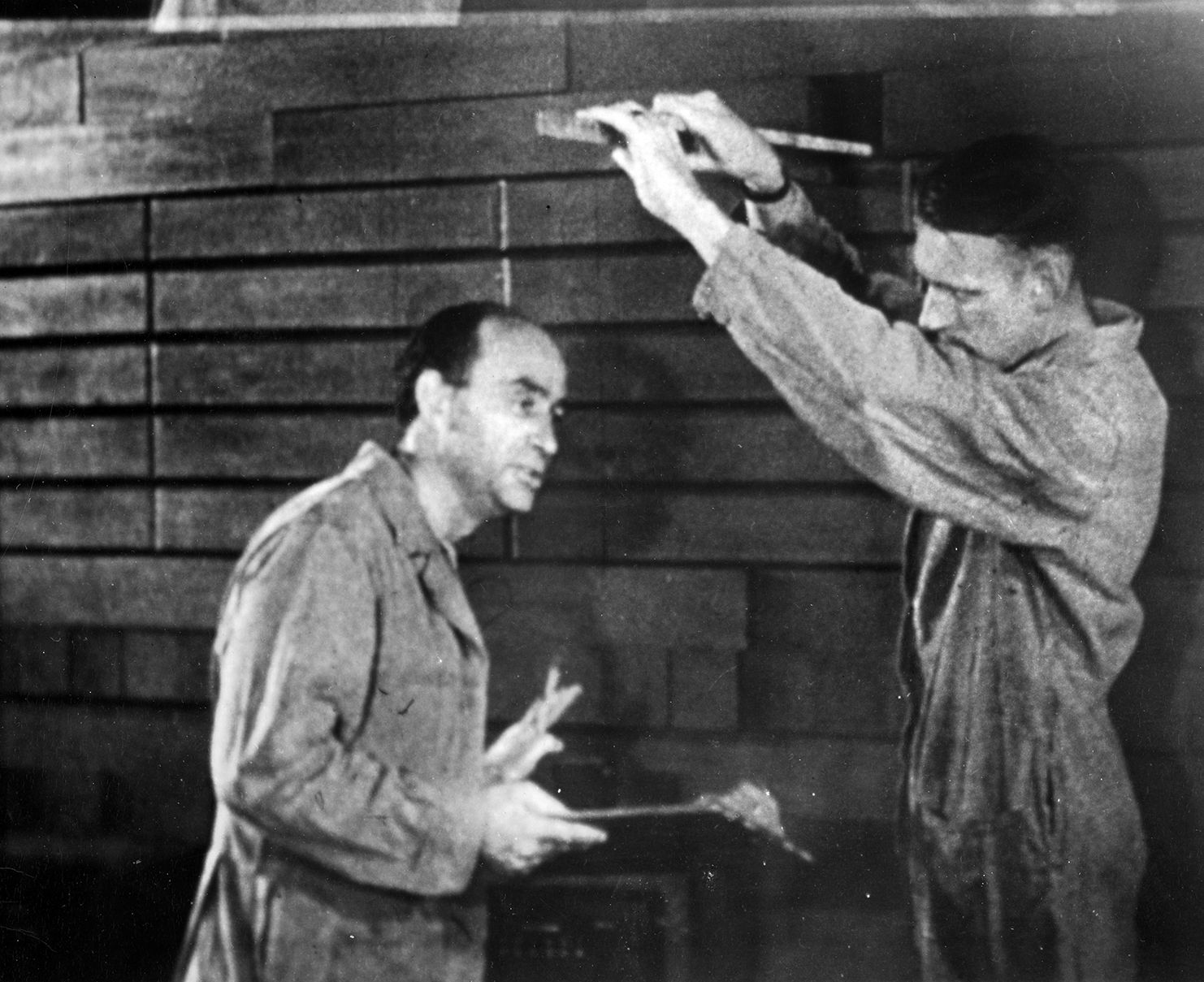
Whenever Fermi decided to accomplish something, he would never give up until his goal was achieved. He spoke English, but with a very heavy, almost stereotyped “just arrived” Italian accent. This linguistic state of affairs was unacceptable to him. One summer in Ann Arbor, Michigan, he insisted a younger colleague endlessly repeat a long sentence that included the dreaded “r” sound like “Rear Admiral.” He also read all the Americana he could and devoured American comic strips, good sources for current lingo and contemporary slang. Alas, the Italian accent remained.
Originally the reactor, which was called a “pile” at the time, was scheduled to be built on a 1,025-acre government plot in the Argonne Forest near Chicago. But difficulties arose, including a labor dispute, so the location was quickly changed to the University of Chicago. Chicago Pile 1, or “CP-1” for short, found a home on the University of Chicago campus. Stagg Field was an abandoned football stadium, shut down when the university administration considered collegiate sports to be too disruptive to traditional academic pursuits.
The specific location was called “West Field,” a curious spot that was part stadium bleacher/stands, part building that was done up in a medieval revival style. On one side, an ivy- covered “fortress” arose, complete with rounded turrets and saw-toothed, crenelated battlements. Yet the opposite side featured tier after tier of spectator stands, an uneasy amalgam of present and mock past.
Though the stadium itself had been abandoned and was ultimately demolished in 1957, in 1942 West Field housed a chamber 60 feet long, 30 feet wide, and 26 feet high that seemed custom built for a reactor. It was used as a squash court, a game reminiscent of racquetball, and also occasionally for handball. Ironically, Leona Woods, the youngest and only woman on Fermi’s team, had played squash there in 1940 as a student. Since it was designed for vigorous exercise, the room was unheated.
Enrico Fermi organized his workers—the ones who were doing the physical labor—into two construction crews, so the work could continue around the clock. It was discovered that graphite, a crystalline form of carbon, could slow down the speed of neutrons and actually promote fission. The reactor “pile” was for the most part row after row of graphite “bricks” arranged in a particular pattern, until Fermi’s precise calculations had deemed the ever-growing stack was high enough.
Eventually the Italian physicist’s number crunching told him that a nuclear chain reaction would be achieved if the reactor stood between 56 and 57 layers tall. Accordingly, the work halted when the crews finished the 57th row. The outermost graphite bricks were solid chunks of graphite, but the ones nearing the center of the pile had holes drilled into them, and those cavities were filled with five-pound “slugs” of uranium. Altogether 18,000 uranium slugs were buried in the reactor, each lump capable of shooting neutrons in all directions.
These graphite bricks were cut and shaped by a team of skilled mechanics under a professional millwright named August Knuth. Their facility was also located at West Field, and altogether 45,000 bricks were made. A lathe was used to cut the holes for the control rods (explained later) and the pockets to hold the uranium. Graphite is the same substance used as “lead” in pencils, and can be dirty. Soon graphite dust filled the air and left a slippery film on the floor.
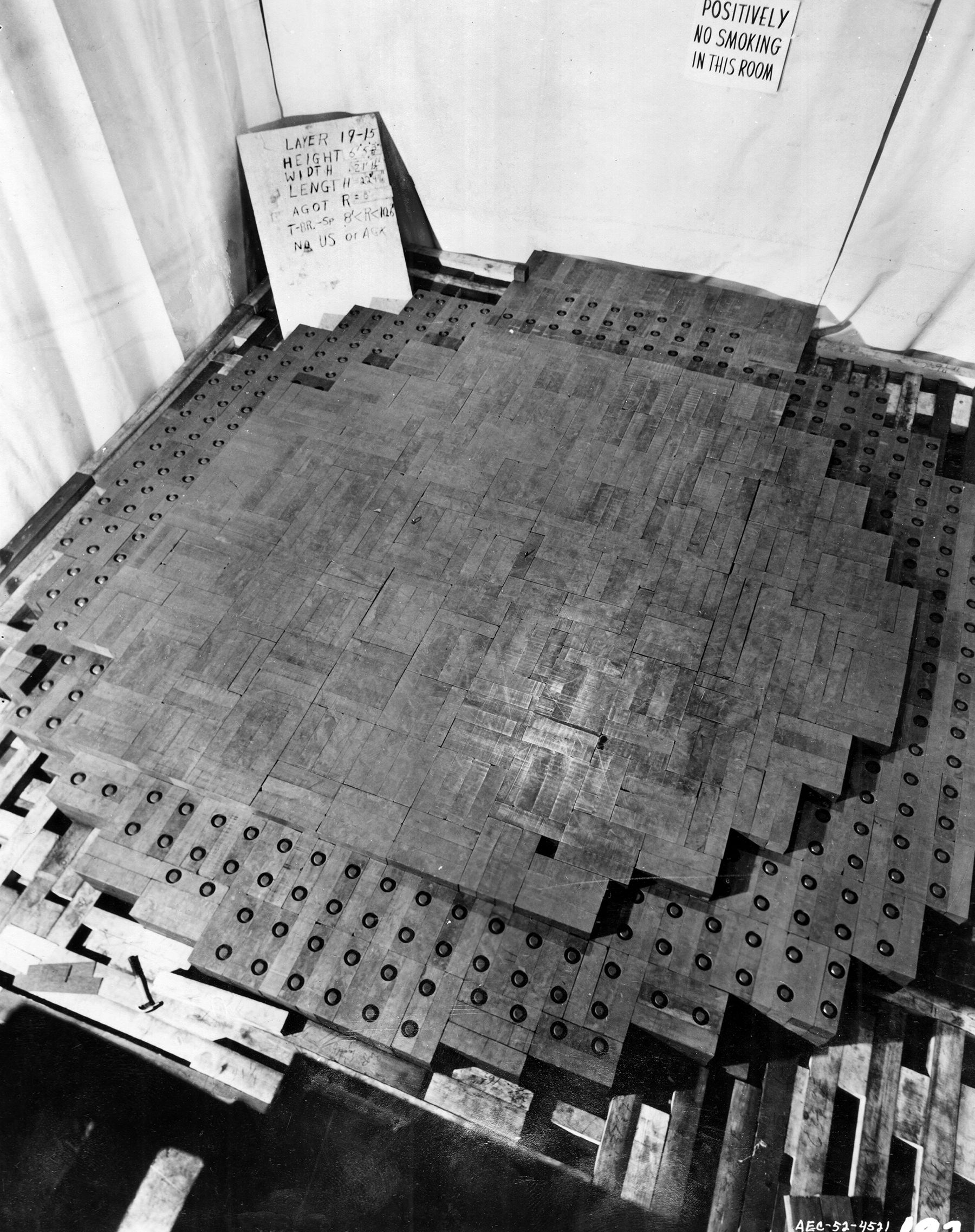
The work was so intense the laborers worked up a sweat, and some removed their shirts—to their later discomfort. Their heads and bodies became so encrusted with graphite they were blacker than coal miners, and even after showers graphite particles would emerge from every pore.
The Goodyear Tire and Rubber Company, known at the time for their association with lighter-than-air “zeppelins,” got an order to make a rectangular cloth balloon for the project. Since the reactor was top secret, they were not given the reason why the government wanted such a strange, aerodynamically crazy thing, but they dutifully complied. This balloon was like a curtain draped on three sides but pulled back like an awning on the fourth side to allow Fermi and his team to do their work.
The CP-1 “pile” was finished on December 1, 1942. A wooden frame supported an elliptical, some said egg shaped, structure. The reactor was 20 feet high, six feet wide at the ends, and 25 feet wide at the middle. The top of the structure, with its rows of graphite bricks stacked in various heights, made it look like a Babylonian ziggurat of ancient times. The pile had holes drilled into it for control and safety rods. These rods were long wooden strips coated with sheets of cadmium, a neutron absorbing substance. Safety was paramount; if the projected chain reaction went wild and control rods failed, the pile might melt down and flood the room with radiation.
When working with such materials, Fermi knew he had to expect the unexpected, and redundancy would boost the scientists’ confidence. There were actually three sets of control rods. Rod one could be raised and lowered via a small electric motor connected to a switch on an instrument panel in the squash court balcony. When the test occurred, that was where the instrumentation as well as most of the scientists would be.
The second rod would be the emergency safety rod. When the time came, it would be suspended over the pile and tied by rope to the balcony. A second rope was tied to the other end of the rod, then strung through a hole bored through the reactor before being tied to some weights. A scientist named Norman Hilberry stood at the ready, axe in hand, to chop the first rope if a meltdown was imminent. Once released, gravity would do the rest, and the safety cadmium rod would plunge downward to absorb the raging neutrons and stop the threatening meltdown in its tracks.
The third rod was the main controlling rod, called the “vernier rod,” which at the moment was buried deep within the dormant atomic pile. It was to be operated by physicist George Weil. It was called a vernier rod because it had markings, a bit like a huge “ruler,” that showed the inches and feet still in the atomic pile.
This vernier rod was the ‘dam” that, at least for the moment, held the neutron chain reaction in check. On Fermi’s orders, based on precise measurements, Weil would withdraw the rod step by step and begin the chain reaction. The movement of the rod was like the opening of a dam’s sluice gates, only instead of water it would be a flood of rampaging, atom-colliding neutrons.
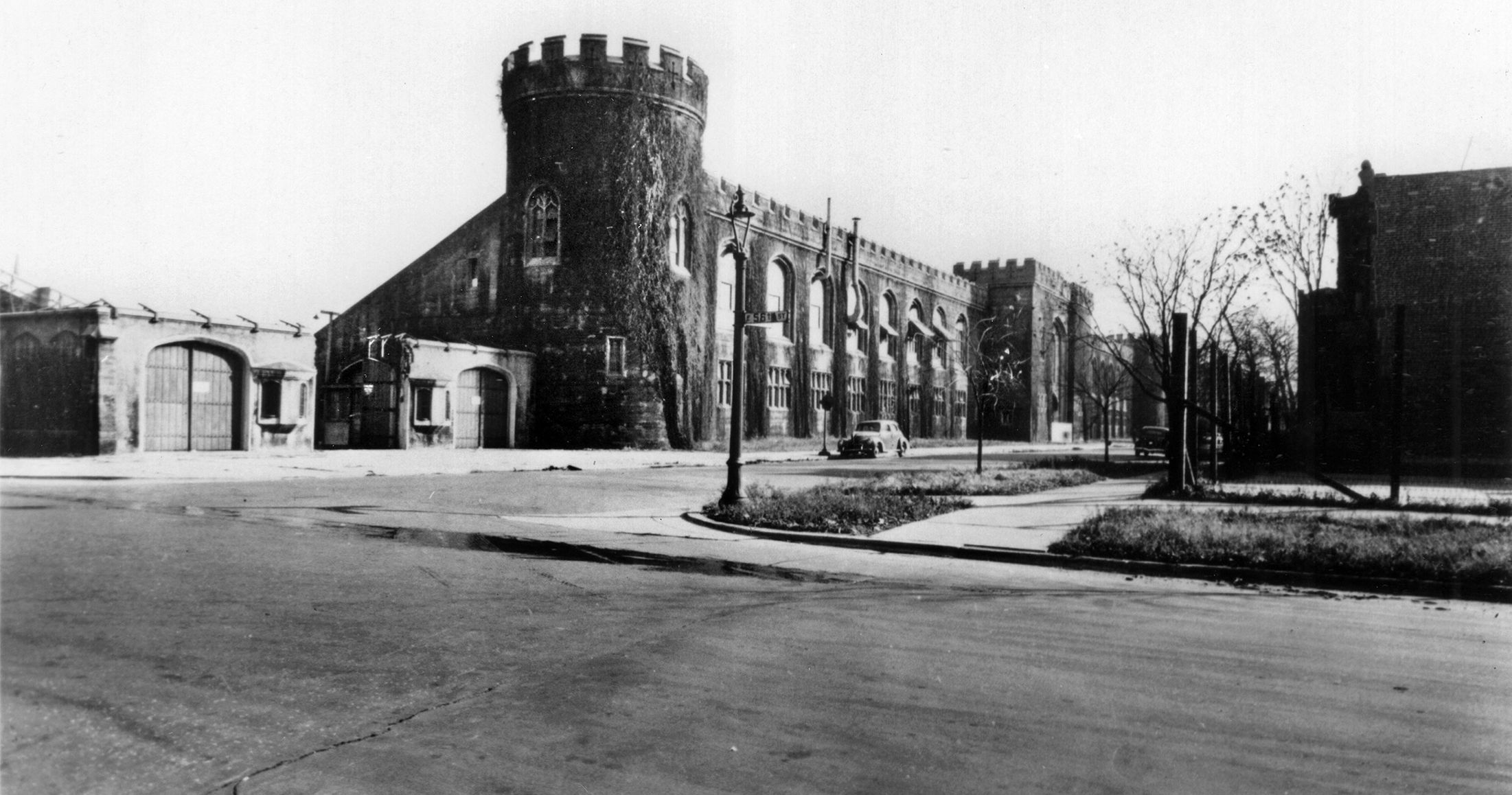
Wednesday, December 2, 1942, was the date set for the make-or-break experiment. It was bitterly cold, with frigid winds coming off Lake Michigan, but even the adverse weather could not mar the scientists’ growing sense of excitement. Altogether 48 individuals were present for this historic event, 47 men and one woman, Leona Woods. Most were assembled on that squash court viewing platform/balcony that overlooked the atomic pile.
At 9:45 a.m., Fermi ordered the withdrawal of the electrically operated control rod. A button was pushed, the rod’s motor whirred, and all eyes were drawn to the panel where lights indicated the progress of the withdrawal. But more attention was soon given to a nearby instrument that measured the level of neutron activity. Its pen sprang to life, quivering and shaking as it recorded rising levels of neutron activity on a slowly scrolling piece of graph paper.
So far, so good. “Zip out!” Fermi declared, meaning the removal of the emergency control rod, and Walter Zinn promptly obeyed. Now, only the vernier rod was left still embedded in the atomic pile. Fermi, his eyes darting from instrument panel to instrument panel, called, “Pull it to 13 feet, George,” and Weil promptly obeyed. The effect was dramatic, as the instruments showed the neutron activity was rising. Leona Woods was on hand to call and record instrument readings.
Many were encouraged, but Fermi was too busy making further computations to share the elation. His brilliant mind, helped by his handy slide rule and constant instrument readings, was drawing quite another conclusion. Addressing those clustered around him, he declared that the plot of neutron activity would rise, but only for a time, then level off. Fermi even pointed to the exact spot on the graph where this leveling off would take place. Sure enough, a few minutes later activity waned and the graph showed a leveling off of activity. It was the exact spot he had indicated.
The process was repeated several more times, with the rod being pulled out at various lengths, which kicked off rapid neutron activity, only to sputter out and level off once again. Yet Fermi was on top of things when the others could only speculate, worry, and wonder. He predicted each leveling off with unerring accuracy because he knew there would be a series of plateaus before the desired chain reaction would be achieved.
Suddenly, a very loud thump burst through the room, shattering the scientists’ concentration and their nerves. The automatic safety rod had crashed down, but it was realized there was no real danger, at least not yet. It was supposed to intervene when the neutron count got dangerously high, shutting down the reactor and saving lives. It seemed the trigger point for activation was simply too low; it was adjusted.
Harold Lichtenberger, W. Nyer, and A.C. Graves stood on a platform over the pile, a group dubbed the “liquid control squad” but nicknamed the “suicide squad.” Armed with buckets of cadmium-laced water, they would be the last-ditch safety measure if all the other safety controls failed. In such a crisis scenario, with an out-of-control chain reaction threatening to release large amounts of radiation into the room, these men would quench the nuclear “fire” by emptying their buckets directly onto the pile.
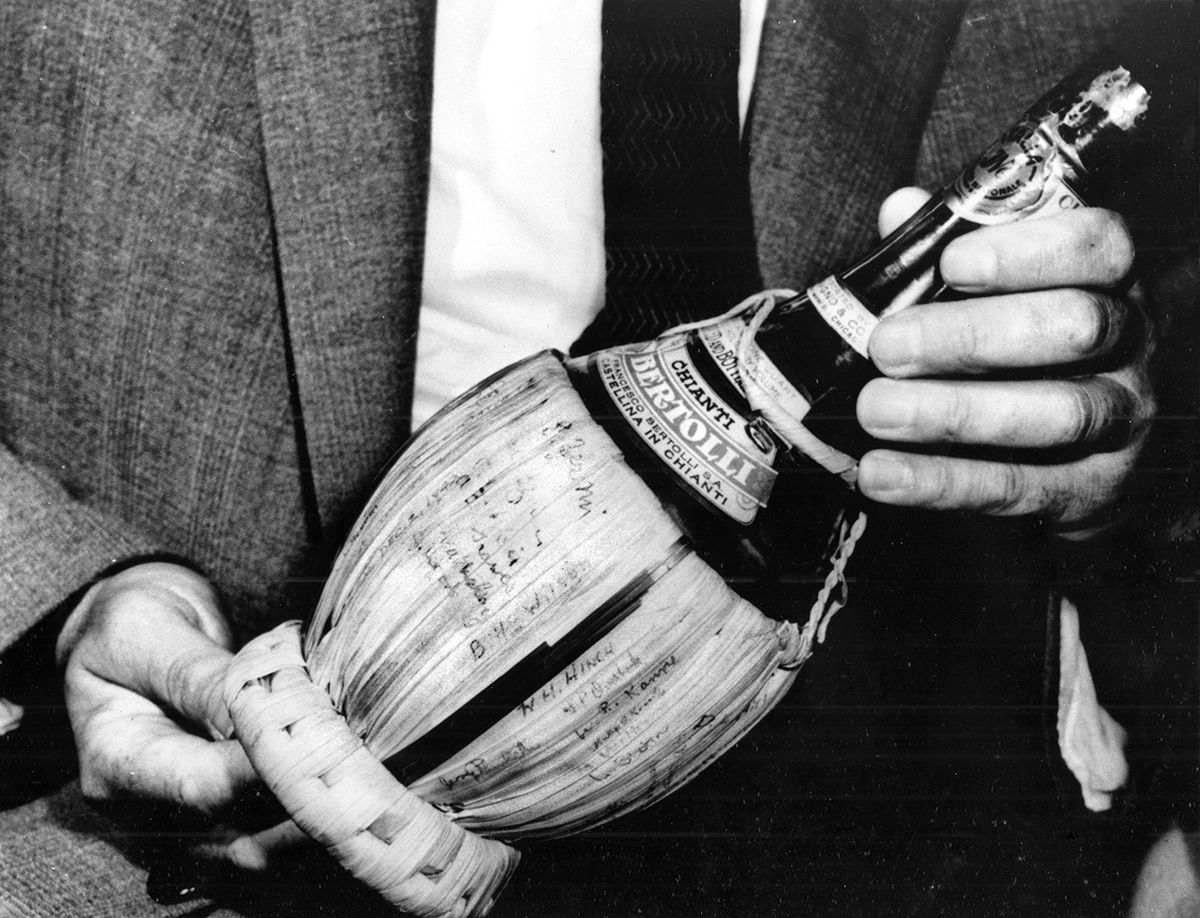
Fermi looked at the relieved faces of his assembled colleagues and rightly figured they needed a break. “I’m hungry,” Fermi declared, “Let’s have lunch!” Distance was always a factor when seeking out lunch—long walks through a cold and often snowy campus, or through the streets of Chicago, was an ordeal best left to the natives. There was only one nearby lunch counter, but one scientist recalled it was a gastronomic ordeal. The “old lady” behind the grill turned out hamburgers that were “tough as shoe leather.”
The team returned to the squash court by 2 p.m. Once again George Weil manned the control rod. “All right, George,” Fermi ordered, and the rod was withdrawn. At 2:50 the rod came out another foot, and the counters moved so fast they nearly jammed, and the clicking they produced sound like the staccato chatter of a machine gun.
Once again, Fermi was calm, the eye in a hurricane of rising expectations. The Italian physicist knew that they were not where he wanted them to be—at least not yet. But they were close. The rod was moved six inches more and then another foot. “This is going to do it,” Fermi firmly declared. They had reached their goal—the pile could officially be called a reactor. “Now it will become self-sustaining,” Fermi continued, “the trace will rise and continue to rise, and will not level off.”
Fermi was carefully watching the rate of rise of neutron counts over a minute span. Once again, he did calculations on his slide rule. Leona Woods and another scientist named William Sturm were recording the data from instruments, with the counters clicking so rapidly individual clicks were beyond the range of the human ear. Suddenly, Fermi’s serious, almost grim face broke out into a broad smile. It was done. At 3:53 p.m. on the afternoon of December 2, 1942, the atomic reactor—and now it could be called a reactor—achieved sustainability.
The reactor didn’t shudder or glow, or do anything spectacular, but nevertheless at that moment the nuclear age was born. Fermi allowed the new reactor to run some 28 minutes, to make sure this was no fluke, but all was well. “OK, zip in!” he ordered and the emergency rod was inserted into the reactor. Immediately the graph pen slowed, then stopped, and the “click” sounds on other instruments were dramatically reduced. It was over.
Hungarian scientist Eugene Wigner produced a bottle of chianti and gave it to Fermi. The Italian uncorked the bottle and poured some wine into waiting paper cups. There was no toast, just sipping the wine in quiet contemplation. The actual atomic bomb would not be produced for another three years, but no doubt many of the assembled scientists were looking ahead, beyond the immediate concerns of the war. The nuclear “genie,” full of danger and promise, had just been released, and the age of anxiety had begun.
Author Eric Niderost is a frequent contributor to WWII History on a variety of topics. He resides in Union City, California, where he is also a college history professor.
Join The Conversation
Comments
View All Comments