By Mark Carlson
There is no disputing that radar played a major role in the Allied victory in World War II. The story of how radar came to be, and the huge effort made to develop it in Great Britain and the United States is hardly known outside of the exclusive fraternity of brilliant scientists and engineers who pulled off one of the most crucial accomplishments of the conflict.
In the summer of 1940, a 29-year-old Welsh engineer named Eddie Bowen was traveling across the Atlantic to the British embassy in Washington, D.C. Next to him in his stateroom, never out of his sight, a small sealed black box held a great secret.
Already considered one of Britain’s most promising physicists, Bowen had been on the cutting edge of radar research since 1935, developing the first crude aircraft radar sets and the invaluable Chain Home Network. Even then, Bowen and his superiors in the government had no illusions regarding the threat of Hitler and the powerful modern air force he was building.
As hordes of German bombers began swarming over England as a prelude to invasion, the Chain Home Network was proving its worth by detecting the enemy planes over the Channel in time for RAF Fighter Command to intercept them. Bowen was named as the newest envoy of a Technical and Scientific Mission to the United States. Inside the box were 12 objects, each small enough to fit in the palm of a hand. They resembled clay pigeons but held the future of Great Britain’s struggle against Nazi tyranny in Europe. When Bowen reached the British embassy, he was met by Sir Henry Tizard, the Director of the prestigious Imperial College of Science and Technology and chairman of the Committee on Air Defense. Tizard was one of Britain’s scientific visionaries. In 1936, he pioneered the early use of Radio Direction Finding (RDF), the predecessor of radar. Knowing of the United States’ industrial and scientific capacity, Tizard’s goal in 1939 was to create an exchange program to share and develop several inventions and theories in military defense.
Even though America was still neutral, there would be advantages in helping Britain improve the new science.
Known informally as the “Tizard Mission,” the team Sir Henry was assembling on both sides of the Atlantic was devoted to improving and utilizing radar to defend England against air attack. The objects Bowen had carried from besieged Britain under great security were the newest design of the Resonant Cavity Magnetron, or RCM, the super-secret and sophisticated core of the rapidly advancing technology of micrometer radar.
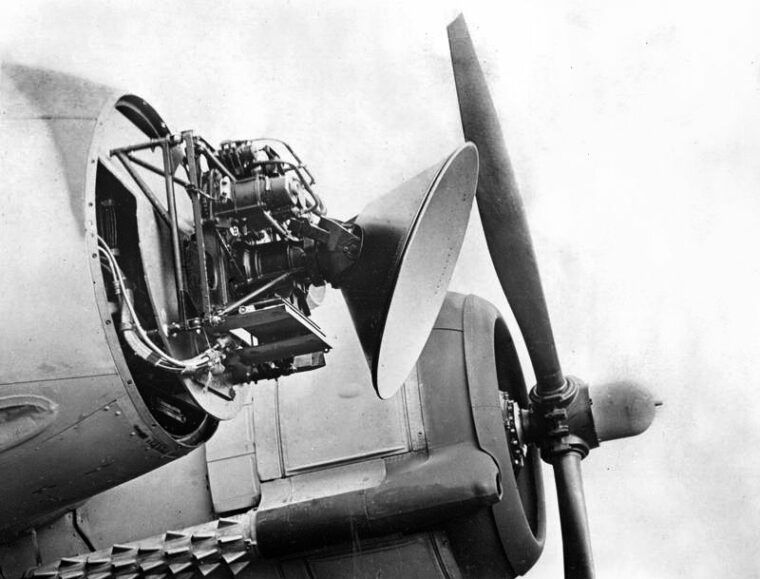
The Cavity Magnetron had been invented only eight months earlier by two physicists at the University of Birmingham. The reasons the RCM was so vital to Britain’s survival were its compactness and power. Placed inside the nose of an RAF fighter, its 10-centimeter pulse would be sharp, losing little concentration as it made its way to an enemy aircraft and back. Longer radio waves, a meter or more, can only detect large areas, such as land features, but a micrometer beam can pick out smaller objects, like aircraft and submarine periscopes.
From the start, the British effort had been in finding a transmitter tube powerful enough to transmit a reliable micrometer-wave radar pulse. The shorter a wave is, the more power it needs to emit a coherent beam capable of traveling miles to a target and back. The RCM was the key to making the micrometer radar work. But there were problems, such as uneven power distribution. With Great Britain shuddering under the Luftwaffe’s bombs and the drain on limited resources, the team could not be sure of a breakthrough before time ran out. Production was another limiting factor. Only the U.S. could manufacture the devices in the numbers needed for war production. Tizard met with Secretary of the Navy Frank Knox to work out the details of the Anglo-American project. He then went to the White House to talk with President Franklin Roosevelt.
That was where Vannevar Bush of the National Defense Research Committee came in. Appointed by President Roosevelt in July, Bush was the most influential member of the NDRC. Bush oversaw every significant scientific and technological program in the country, the much-needed bridge between the mutually distrustful civilian scientists and the old guard military. He gathered the brain trust of Stanford, Harvard, Columbia, Yale, the Massachusetts Institute of Technology, and the National Academy of Sciences, as well as physicists from the University of California Berkeley. Another prime member of the NDRC was Bell Labs, whose pioneering work in communication and radio were to be valuable. MIT would head up the radar team.
From there, the next step was to test the device and determine its power. Financier and scientist Alfred Loomis had already put together a private research lab at his estate in Tuxedo Park, north of New York City. This is where Bowen met with the radar pioneers. On Sunday, September 29, 1940, Bowen carefully revealed one of the diminutive RCM units. Loomis and other members of the team were there to witness the unveiling. They marveled at the small object that might be the key to winning the war.
The first real test of the RCM on American soil took place at the Bell Laboratories on Sunday, October 6, the same day Hitler ordered nighttime bombing of London, forcing the city’s inhabitants to sleep in the Underground as their homes burned. With the Luftwaffe bombing Britain at night, the Chain Home Network’s long-wave radar did not possess the resolution for successful interception. What was needed was a micrometer radar that would fit into a fighter. The RCM could be decisive.
Bowen held his breath as he set the magnetic field potential and ran the filament up to the needed level. Then, they switched on the anode. In a moment the assembled scientists were rewarded with a blue glow emerging about an inch from the output terminal. With wide eyes and open mouths, the team stared in wonderment. “I had not seen anything like this before,” Bowen admitted. Initial estimates put the output at about 14 kilowatts, seven times what the Bell Labs own centimeter-wave unit produced. It was a breakthrough. It was almost a thousand times greater than the best micrometer radar in use in the U.S. From that moment on, things moved fast. It had, according to Alfred Loomis, ”pushed the American research in radar ahead at least two years.”
This was exactly what was needed at exactly the right time. Things were not going well across the ocean. In the weeks since Germany began the Blitz, over 7,000 Britons had been killed in Luftwaffe air raids. On September 27, Japan joined Germany and Italy as the third member of the Axis Powers, portending a second major front in the Pacific. A week after the first demonstration of the RCM, a new member joined the team. Summoned from Berkeley by Bush, this was Ernest Lawrence, the Nobel Prize winner from California. He was one of the most brilliant physicists in the country, almost on par with the great Albert Einstein. His invention of the cyclotron would soon open the door to the study of the atomic nucleus, leading to yet another NDRC venture, the Manhattan Project.
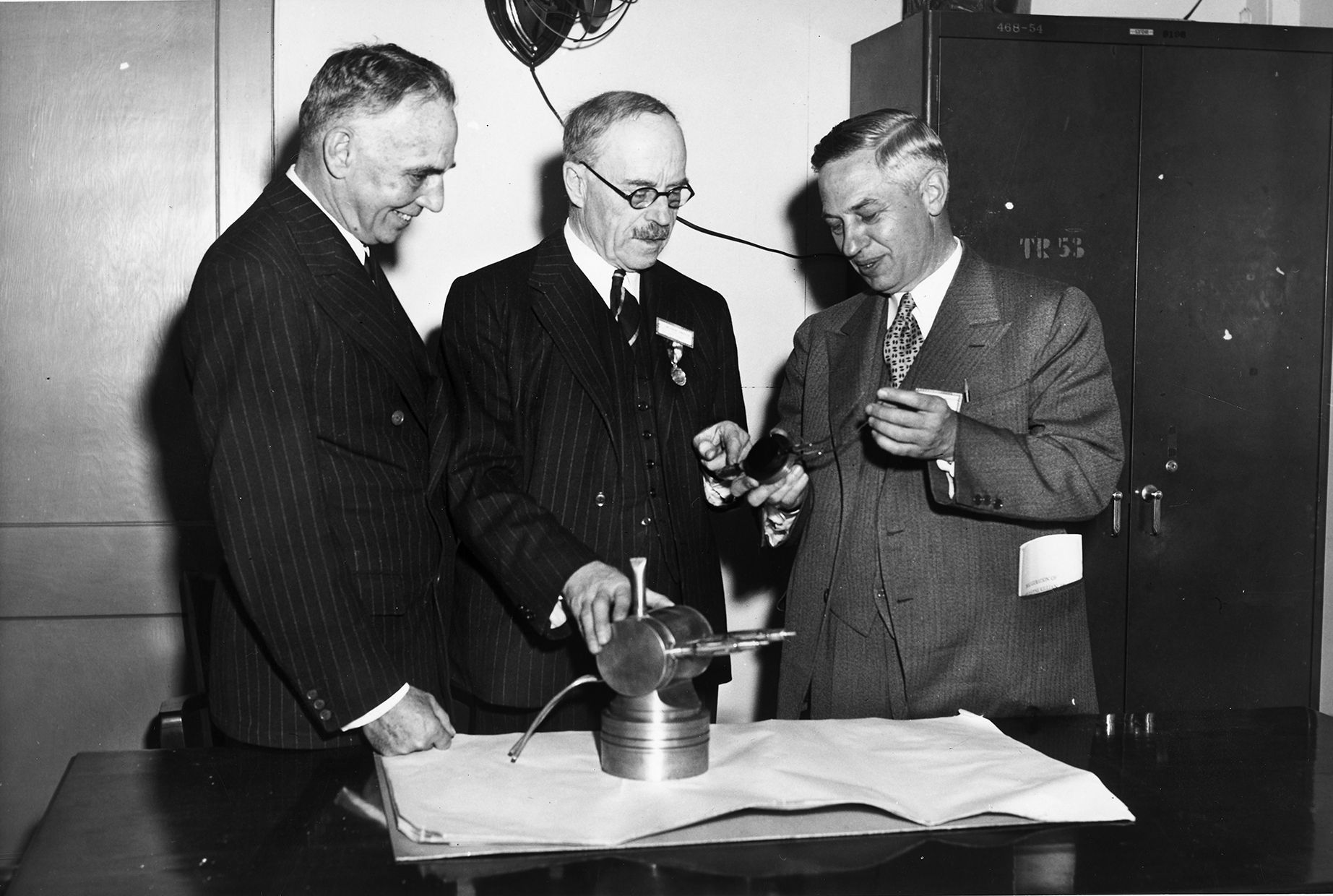
But more was needed than to simply mass-produce the magnetron. Sensitive receivers, new antenna designs, generators, and a myriad of other devices had to be invented specifically for the various services. Antiaircraft, bombing, air intercept, submarine- and mine-hunting, and naval surface radars had to be brought from conception to blueprint to testing to mass-production and deployment to the war zones. The United States, with its nearly limitless resources and funding, was the ideal place to develop radar. Bowen made it clear that the most urgent need was a 10-centimeter radar for night fighters. The second goal was for a radar capable of controlling antiaircraft guns.
Lawrence, who had joined the team with little confidence in England’s ability to survive the Blitz, was won over by Bowen’s pitch. “This changes the odds,” he said. “Let’s get going as fast as possible, and do anything we can to help the British.”
Loomis had not been idle since the first demonstration. He used his considerable clout to add RCA and the Sperry Corporation to the team. Five magnetron sets were needed for initial development. Bell Labs would produce the magnetrons, Sperry the parabolic reflectors, and RCA built the cathode-ray tubes, modulated power supplies and intermediate frequency amplifiers. General Electric manufactured more magnetrons while Westinghouse joined RCA in producing antennas.
Bush, the NDRC, and the Microwave Committee agreed to set up a new research and development laboratory on the grounds of MIT on October 18, 1940. The speed at which this was done was an indicator of the importance of the project. Officially it was called the Radiation Laboratory to disguise its true function. In 1940, no one took the military use of nuclear research very seriously. The facility was called the “Rad Lab” by its members.
According to Carl Compton of MIT, the Rad Lab “…was the greatest cooperative research establishment in the history of the world.”
Bush and Loomis secured hangar space at East Boston Airport for the airborne-intercept project. The Rad Lab, which had adopted Bowen right off, nicknaming him “Taffy” from his Welsh roots, went right to work. Dozens of physicists and engineers were recruited from all over the country. About 20 were brought into the exclusive microwave fraternity after having been sworn to secrecy. There was no shortage of talent. The Rad Lab was the place to be.
Among the assembly was Lee A. DuBridge from Rochester, where he had built the university’s first cyclotron. He would eventually become the Rad Lab’s director. DuBridge wrote to Lawrence, “I’m still dizzy at the pace things are going. Everyone is working together beautifully, and it’s going to be great fun.”
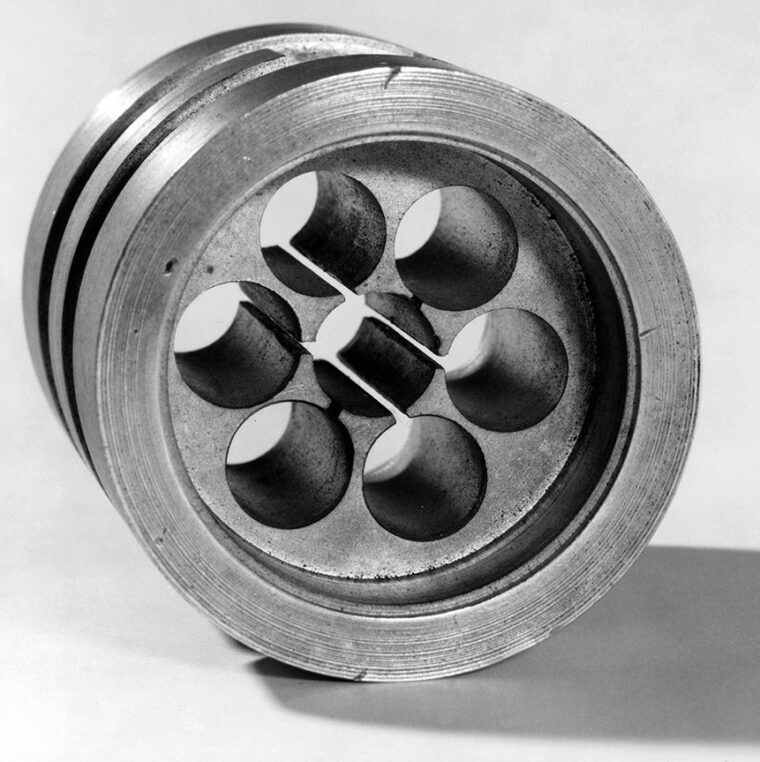
The projects with the highest priority were an aircraft-mounted interception radar, an antiaircraft interception system, and a radar to enable bombers to hit targets through dense clouds. These were brought together under the name THOR, a reference to the God of Thunder who could destroy his enemies at a distance with lightning bolts.
The difficulty in seeing, tracking and training an antiaircraft gun toward a distant, fast-moving aircraft was virtually a hit or miss situation. It was too much to expect a human gun crew to rapidly swing and elevate a heavy gun along an aircraft’s flight path and fire shells to explode at the same altitude where the plane would fly into it. Radar did alert a AAA battery to the enemy plane’s altitude and distance, but it still required human reflexes and muscle to move the heavy gun into position. What was needed was a true gun-tracking system, one that could not only pinpoint the target, but also move the gun and track it along its flight path – in short, a fully automatic fire-control system. While the navy did have the superb Mark 37 Fire Control System for secondary (5-inch/38-caliber) guns that kept the turrets and guns on a moving ship on target, it was a far cry from a radar that could do the same thing with a fast-moving aircraft. Only the new microwave radar was up to the task. But how to translate an electronic target blip into mechanically directed fire control?
One of the most critical elements came into use just after the D-Day invasion of Normandy on June 6, 1944. German engineers had built and deployed the first of the so-called Vengeance Weapons, starting with the V-1 “Buzz Bomb,” a 27-foot-long pilotless ram-jet missile that could reach from France to London. The Buzz Bomb was launched from rails and flew in a straight line to crash and detonate nearly a ton of Amatol explosive on whatever target happened to be below. With an airspeed of more than 320 knots, it was too fast for propeller-driven planes to catch. But with radar, the RAF and USAAF were able to intercept and dive on the bombs, shooting them down in sparsely populated areas. The problem was shooting down enough of them. The new SCR-584 mobile radar units became the first part of the Rad Lab’s technological triad that would become the terror of Axis aircraft.
The SCR-584 had first been tested in May 1941. It made use of the tight microwave radar pulse from the RCM with far more accuracy than the previous SCR-270 units that were already in service. One of the more infamous 270 units was on OPana Point on the northern tip of Oahu on the morning of December 7, 1941. While the unit did detect the incoming Japanese strike force, it was human error that had failed to recognize the threat. Another SCR-270 was on Midway on June 4, 1942, and pinpointed the first wave of Japanese bombers coming to hit the island. In both cases, the longer-wavelength 270s were able to establish a rough range and azimuth, but not altitude or composition.
When the 584 was first tested it was almost a quantum leap forward in pinpointing range, azimuth and altitude. But like its predecessor, the 584 could only detect the enemy, not shoot them down. The next step was what became known as the Bell Labs Mark 9 Predictor.
Basically an early analog computer, the M9 was conceived by David Parkinson of Bell Labs. He designed a system that, upon detecting a moving target, would traverse the antenna’s cone-shaped beam to keep the target in the center of the radar beam. In other words, the antenna tracked the aircraft. The M9 was an analog computer able to compute where to fire a shell to explode just when the plane intersected it. From there the M9 sent its data via cable to the AAA batteries to automatically swing and elevate the gun to fire and intercept the target.
The final piece of the triad was the proximity fuze. Prior to its invention, antiaircraft shells were detonated by altimeter, time fuze or impact. Of course all of these depended on precise calculations or random chance to work. During the London Blitz, it took roughly 20,000 shells to bring down a single plane. Other calculations put it as much as five times higher. The proximity fuze was the brainchild of Merle Tube of the Carnegie Institution. Tube was a friend of Lawrence and developed pulse radar. Pulse radar was an important step toward designing an influence fuze to detect a nearby aircraft. Acoustic, infrared, photoelectric and radio were impractical. The final concept was a compact, continuous wave radar to be screwed into the hull of a standard artillery shell, forming the nose. It would emit a steady oscillating 180 to 220 megahertz signal. The shell itself constituted one electrode of the dipole, with the fuze itself as the other. A plastic nose, transparent to radio waves, covered the receiving antenna. Any metal object that was detected within a few megahertz of the pulse triggered the detonator. The first obstacle was to design four vacuum tubes the size of a fingertip to fit into the fuze and be rugged enough to withstand the shock of firing. Tube created a chemical combination of zinc, carbon and chromic acid, that would, upon firing, cause a galvanic reaction and serve as a battery. By April 1942, the fuze was ready for testing at the secret Applied Physics Laboratory in Silver Spring, Maryland. In order to maintain secrecy, the plastic nose cones were shipped via Johns Hopkins University Hospital as “rectal spreaders.”
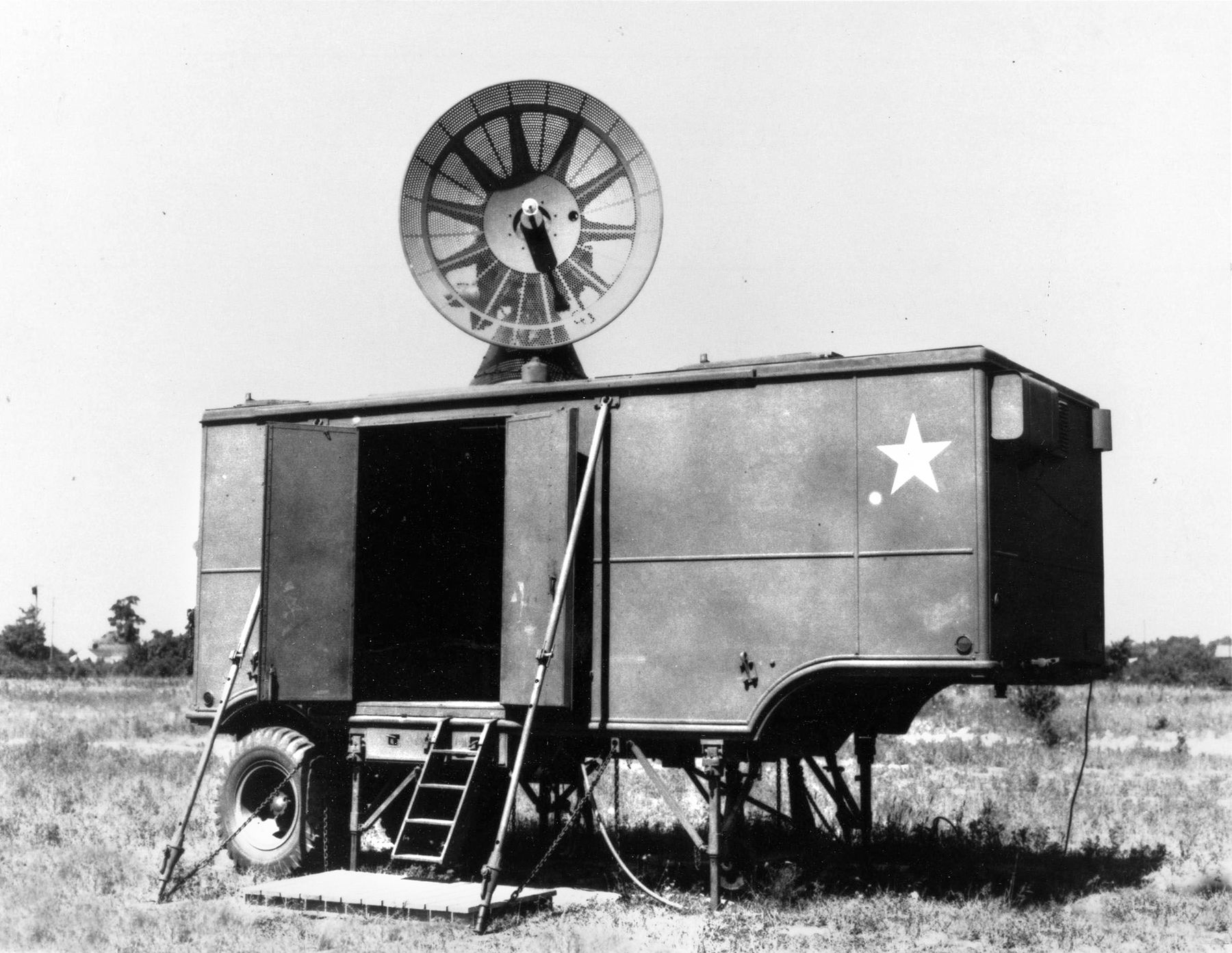
After a successful test series, Sylvania Corporation began producing the shells at a rate of 400 per day. By late 1944, Sylvania and other companies were mass-producing 70,000 of them a day in various calibers. The deadly antiaircraft triad was complete. With the SCR-584 radar Unit, the M9 Predictor, and the proximity fuze, there was no escape for an enemy plane.
The location of the first deployment of the system was a problem. The V-1 Buzz Bombs coming from German-occupied territory meant setting up AAA batteries in Belgium. But this risked dud proximity fuzes turning up in German hands for examination. That could not be allowed. But a consignment of the new fuzes had arrived in England at the start of the V-1 offensive. Any dud shells would fall into the Channel. Since the Buzz Bombs flew in a straight line, they were easier to shoot down.
At selected points along the south and east coasts of Britain, the SCR-584 units were assembled with a generator nearby. Cables connected the M9 Predictor on its own trailer. Then 90mm guns were stationed at four points about 50 yards away. Rad Lab engineers Leo Sullivan and Lee Davenport trained the gun and radar crews. By the end of August, the guns and fighters were bringing down about 70 percent of the Buzz Bombs. On August 28, the last day the Germans launched the V-1s before the launching sites were overrun by Allied troops, 104 were launched, of which the SCR-584 AAA guns shot down 64, while fighters accounted for 14. Only four reached London, the rest having crashed.
In all, the antiaircraft batteries shot down 1,600 V-1s—about the same as the fighters. The 584 had been one of the Rad Lab’s biggest gambles, but turned out to be its greatest success. It saw action in Europe after D-Day, when army artillery troops learned to use the system and the proximity fuze on German tanks and gun emplacements. They were so effective that even at night the Germans were forced to retreat from the deadly accurate fire of the 584’s invisible radar. Many surrendered and ran to the Americans, yelling “Kamerad!”
Prisoners called the proximity fuze cannonade the most “demoralizing thing they have ever endured.”
The next theater for the new system was the Pacific, where the Kamikaze threat was killing ships and men in horrifying numbers. As the Allies moved north toward the Japanese Home Islands, the numbers would only grow worse. The most remarkable use of the 584 was first demonstrated in early 1944, when General Douglas MacArthur was moving up the north coast of New Guinea. Rad Lab engineer Henry Abajian was present as the first 150 sets were deployed to the U.S. Army’s antiaircraft batteries. But he found the veteran gun crews to be uncertain of the 584’s effectiveness. “They set up the batteries as if they still had to depend on optical gun-laying,” he said. “They were ignoring the automatic tracking.”
Abajian and a General Electric technician toured New Guinea, Fiji, New Georgia, and the Green Islands area, training the crews. Abajian arrived on Leyte in the Philippines during the heavy bombing by Japanese planes after the Allied landings in October 1944.
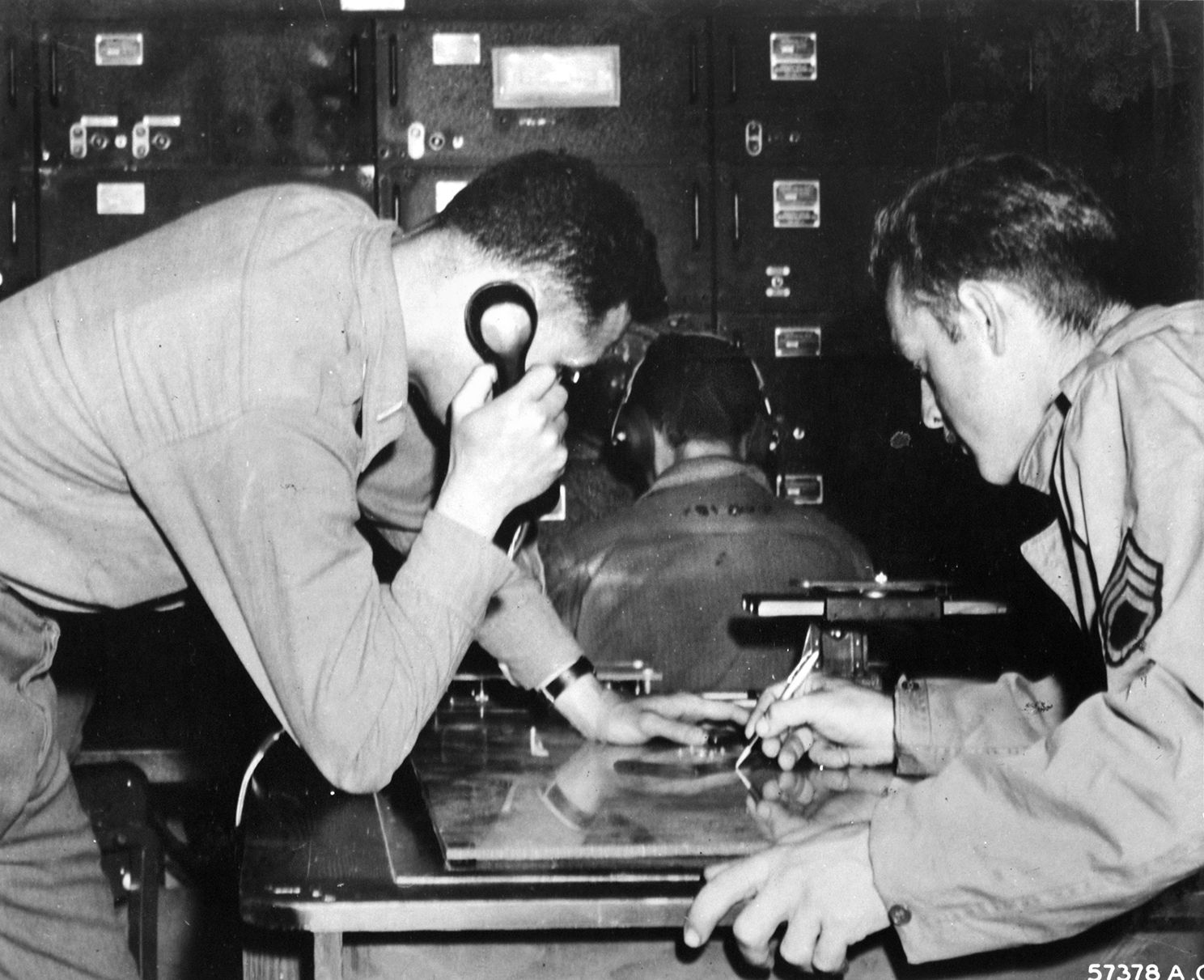
The first six radar units went ashore immediately behind the first wave of assault troops. Over the next two weeks, the 584-directed antiaircraft guns brought down about 300 Japanese planes. “Superior shooting,” MacArthur said in a message to the AAA regiment commander. But those kills were done without the full potential of the system. Abajian went to Mindoro, south of Leyte, where he visited four 584s controlling 16 90mm AAA guns. “They were still not using the system the way it had been designed.” In order to convince the battery commander of how effective the full system was, Abajian connected a set of searchlights to a spare 584.
“And when we turn on those lights,” Abajian said to the officer, “I guarantee there will be an aircraft in that beam.”
The officer agreed, but the searchlights would only be illuminated after the guns had fired, so the lights would not alert the pilot. When the next plane was detected by the 584s, The Rad Lab engineer waited as the commander spoke to his gunners. “‘A’ Battery locked on target. Fire when ready.”
“Commencing fire!” the gunners shouted.
The 16 big guns roared at once, strobing the night sky like brilliant fireworks. At that moment Abajian switched on the searchlights. “Sure enough, right in the center of that beam was this Jap bogey,” he remembered. A second salvo burst out, and the plane was turned into a bright yellow and orange meteor as it fell to earth. The searchlights followed the dying plane all the way down.
Cheers erupted from every man in the battery. There, on that dark night in the Philippines, the feverish work of Bowen, Tizard, Bush, Lawrence, DuBridge, Parkinson, and 1,000 others was vindicated in the most dramatic way. From that moment on, no one would doubt that radar was going to win the war.
Author Mark Carlson has written on numerous topics related to World War II and the history of aviation. He resides in San Diego, California.
Join The Conversation
Comments
View All Comments